Comprehensive Analysis of Vertical Farming: Goals, Contradictions, and Investment Requirements
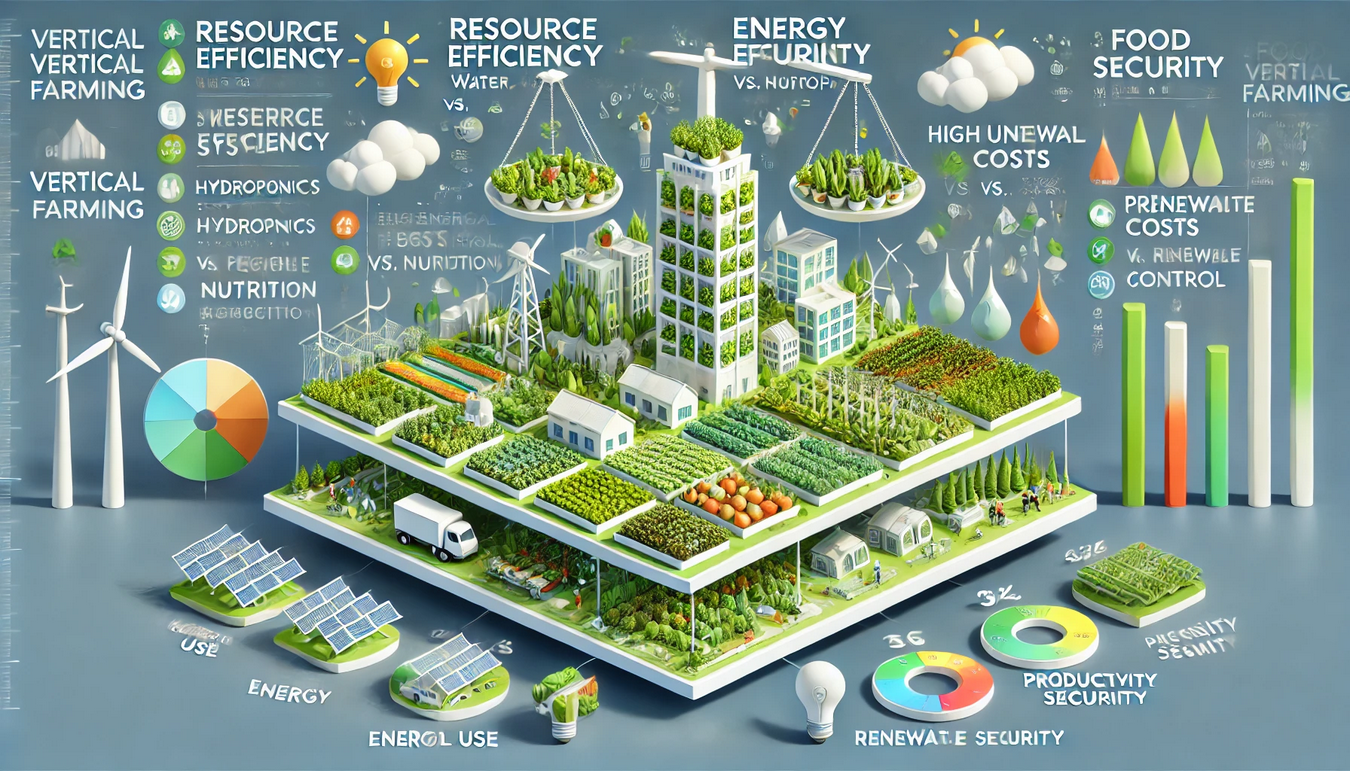
Introduction
With the global population projected to reach nearly 10 billion by 2050, coupled with increased urbanization, the demand for sustainable and efficient food production systems is greater than ever. Traditional agriculture faces numerous challenges, including limited arable land, water scarcity, and the growing impacts of climate change. Vertical farming (VPS) is a promising solution that seeks to produce food in controlled environments, using less land and water while maintaining high efficiency and product quality. The document “Vertical Farming Goes Dynamic: Optimizing Resource Use Efficiency, Product Quality, and Energy Costs” outlines the key objectives, contradictions, and financial requirements for the implementation and expansion of vertical farming systems. This post provides an in-depth academic analysis of the goals outlined in the document, the inherent contradictions in the system, and the required investments for scaling vertical farming.
Goals of Vertical Farming
1. Resource Efficiency
Maximizing resource efficiency is a central pillar of vertical farming. Traditional agriculture consumes vast amounts of water, land, and nutrients, while VPS offers a controlled environment that reduces the overall resource footprint. The main resource efficiency goals include:
- Water Efficiency: VPS systems such as hydroponics and aeroponics can reduce water usage by up to 90% compared to traditional farming, due to their closed-loop irrigation systems that recycle water. This efficiency is particularly important in regions experiencing water scarcity, where agriculture is a major contributor to freshwater depletion. By minimizing water usage, vertical farming not only contributes to sustainability but also ensures a steady supply of fresh produce even in arid regions. Water recycling in VPS also helps mitigate the environmental impact associated with conventional agriculture, where runoff from fertilizers can pollute local water bodies.
- Land Use Efficiency: By growing crops in vertically stacked layers, VPS can produce up to 10–60 times more yield per square meter than conventional farming. This efficiency is crucial in urban environments where space is limited, allowing food production to be integrated into urban planning. By utilizing underused spaces such as rooftops, old warehouses, and other buildings, VPS can contribute to local food systems, reducing the dependence on long supply chains and minimizing transportation-related emissions. The potential to produce food closer to the consumer also reduces food waste associated with spoilage during transit.
- Nutrient Efficiency: VPS ensures precise nutrient delivery directly to plant roots, minimizing waste. Unused nutrients can be recycled back into the system, reducing environmental leakage and improving overall efficiency. Precision nutrient management also ensures that plants receive the optimal balance of nutrients for growth, which can lead to increased productivity and better crop quality. By eliminating the problem of nutrient runoff, which is a significant environmental issue in traditional farming, VPS contributes to reducing the eutrophication of nearby water bodies and promotes more sustainable agriculture practices.
2. Energy Efficiency and Cost Optimization
Energy use, particularly for artificial lighting, is a critical factor in VPS. Given the energy-intensive nature of vertical farming, improving energy efficiency and reducing costs are key objectives:
- Artificial Lighting: LED technology is commonly used in VPS due to its energy efficiency and ability to provide customizable light spectra tailored to plant needs. The document emphasizes the importance of dynamic light management, which optimizes light intensity and spectrum based on plant growth phases and electricity prices to minimize energy consumption. LEDs not only save energy compared to traditional lighting systems but also enhance crop quality by providing optimal light conditions for photosynthesis. The advancement in smart lighting systems that adjust in real-time to the needs of plants further helps in optimizing energy consumption and enhancing overall system efficiency.
- Renewable Energy Integration: The goal is to transition VPS to renewable energy sources such as solar and wind power, thereby reducing the carbon footprint and long-term operational costs. This shift is also essential for achieving zero carbon emissions by 2050. Integrating renewable energy into VPS is challenging due to the high and continuous energy demands of vertical farms. However, advancements in renewable energy storage technologies, such as battery systems and other energy storage solutions, are gradually improving the reliability of renewable energy. Additionally, hybrid systems that combine multiple renewable energy sources can help meet the constant energy requirements of vertical farms and reduce dependency on fossil fuels.
3. Food Security and Product Quality
Vertical farming offers a solution to food security challenges, especially in urban environments where arable land is scarce. The controlled environment of VPS ensures consistent product quality and year-round production:
- Stable, Year-Round Production: VPS can provide a reliable and predictable supply of fresh produce, regardless of external weather conditions. This helps reduce the impact of seasonal fluctuations on food availability. By insulating food production from unpredictable weather events and climate change impacts, vertical farming can play a key role in stabilizing the food supply and mitigating risks associated with food shortages. This capability is particularly significant in regions prone to extreme weather or where climate change has led to unreliable growing seasons.
- Food Safety and Purity: The enclosed nature of VPS reduces the risk of contamination from pests and diseases, minimizing the need for pesticides and herbicides. This leads to cleaner, safer food products for consumers. The absence of chemical pesticides also contributes to improved environmental health by reducing the release of harmful substances into the ecosystem. In urban areas, where concerns about pollution and contaminants are heightened, the ability to produce pesticide-free, safe food is an important advantage of vertical farming.
- Nutritional Quality: Through precise control of nutrient delivery and environmental factors, VPS can produce crops with enhanced nutritional profiles, potentially increasing the nutrient density and health benefits of the produce. By optimizing factors such as light spectra, temperature, and nutrient mix, growers can tailor their crops for specific nutritional outcomes. This capability can also support the development of functional foods—foods specifically designed to provide health benefits beyond basic nutrition—thereby contributing to better public health outcomes.
Contradictions in Vertical Farming
Despite its many advantages, vertical farming presents several inherent contradictions that complicate the realization of its full potential.
1. Energy Use vs. Carbon Reduction
One of the most significant contradictions in VPS is the tension between its energy demands and the goal of reducing carbon emissions. Although the document highlights energy efficiency and the need for renewable energy, vertical farms are highly energy-intensive due to artificial lighting and climate control systems.
- Dependency on Fossil Fuels: Unless VPS operations are powered entirely by renewable energy, their reliance on grid electricity, which may be sourced from fossil fuels, can lead to a substantial carbon footprint. Achieving carbon neutrality by 2050 requires a complete transition to renewables, which poses technological and financial challenges. In many regions, the current energy grid relies heavily on fossil fuels, making it difficult for vertical farms to achieve their sustainability targets without significant changes in the energy infrastructure.
- Challenges of Renewable Integration: While renewable energy offers a solution, its intermittent availability (due to weather conditions or time of day) and the current limitations in energy storage technology make it difficult to guarantee a constant energy supply. This creates a contradiction between the goal of zero-carbon emissions and the operational needs of vertical farms, which require continuous power. To address this issue, vertical farms are exploring innovative solutions such as microgrids, which combine renewable energy generation with storage systems to create localized, self-sufficient energy sources. However, these technologies are still costly and not widely accessible.
2. Cost Efficiency vs. High Initial Capital Investment
The document underscores the long-term cost-efficiency of VPS through reduced water, nutrient, and land usage. However, the high initial capital investment required to establish vertical farms presents a significant contradiction:
- High Upfront Costs: Building a vertical farm involves substantial costs related to construction, advanced technology (LED lighting, automation, sensors), and climate control systems. The document estimates that setting up a VPS can cost anywhere from $3–15 million per hectare, depending on the level of technological sophistication. These costs are a major barrier to entry, particularly for small-scale farmers and startups who may not have access to substantial financial resources.
- Operational Costs: Even after the farm is established, operational costs remain high, particularly for energy and labor. Energy costs for lighting, heating, and cooling are particularly significant. While operational costs may decrease over time due to energy efficiency improvements, the initial financial burden can deter widespread adoption. Government subsidies, financial incentives, and partnerships with renewable energy providers can help reduce these costs, but they require robust policy support and long-term planning.
3. Productivity vs. Product Quality
While vertical farming systems can accelerate plant growth and increase yield, there is a potential trade-off between maximizing productivity and maintaining high product quality:
- Accelerated Growth Cycles: To maximize yield, vertical farms often use techniques that accelerate plant growth. However, this rapid growth can sometimes result in lower nutrient density or diminished flavor, creating a contradiction between the goals of high productivity and superior product quality. Rapid growth can cause the plants to focus on biomass production at the expense of developing complex flavors or nutrient profiles, which may negatively impact consumer perception of quality.
- Intensive Cultivation: High-density planting and intensive cultivation practices, while increasing yield per square meter, can stress the plants, potentially leading to reduced quality. Ensuring that accelerated production does not compromise the nutritional value or taste of the produce is a key challenge for VPS operators. Balancing the need for high output with the desire to produce flavorful, nutrient-rich food requires careful management of environmental conditions and growth strategies, which can be complex and costly.
Investment Needs: A Numerical Analysis
Scaling vertical farming to meet global food demands and achieving the goals outlined in the document requires significant financial investment. Below is a breakdown of the estimated costs associated with implementing VPS on a larger scale:
- Capital Expenditure: The initial capital expenditure for establishing a vertical farm ranges from $3–15 million per hectare. This includes costs for building infrastructure, installing LED lighting systems, automated climate control, and irrigation systems. These investments are crucial for ensuring the scalability and efficiency of the vertical farming model, particularly in densely populated urban areas where space optimization is critical.
- Operational Costs: Annual operating costs for a vertical farm can range from $150,000 to $500,000 per hectare, depending on energy prices, labor costs, and the level of automation. Energy costs alone can account for 60–85% of operational expenses, particularly in regions with high electricity prices. Implementing energy-saving technologies, such as more efficient LEDs and better climate control systems, can help reduce these costs over time, but the initial investment in such technologies is substantial.
- Revenue Potential: Vertical farms can produce up to 60 times more yield per square meter compared to traditional farming, depending on the crop. For high-value crops such as leafy greens and herbs, the revenue potential can range from $1.5 to $3 million per hectare annually, depending on market conditions. The economic viability of vertical farming is heavily influenced by the types of crops grown, with a focus on high-margin produce being more likely to offset the high operational costs and generate profit.
- Renewable Energy Investment: Transitioning VPS to renewable energy requires additional investment. The cost of installing solar energy systems is approximately $2–5 million per megawatt of capacity, with further investment needed for energy storage solutions to ensure a consistent energy supply. For vertical farms, achieving energy independence through renewables is a long-term goal that requires substantial capital, but it is key to ensuring sustainability and reducing operational costs related to energy consumption.
- Government Support and Subsidies: In many regions, the success of vertical farming depends on government subsidies or incentives to offset the high capital costs and support the transition to renewable energy. Without such support, the financial feasibility of VPS may be limited, especially for smaller operators. Public policy plays a crucial role in enabling vertical farming through grants, tax incentives, and research funding aimed at improving technology and reducing costs. Collaboration between the public and private sectors is essential for creating a supportive environment that fosters the growth of vertical farming initiatives.
Conclusion
Vertical farming presents a revolutionary approach to food production, offering the potential to enhance resource efficiency, improve product quality, and contribute to global food security. However, the system faces significant contradictions, particularly regarding energy use, cost efficiency, and product quality. While the long-term goals of zero-carbon emissions and cost savings are achievable, the high initial capital investments, energy demands, and technological complexities pose substantial challenges.
Achieving the goals outlined in the document will require substantial investment in both infrastructure and renewable energy sources. Additionally, overcoming the contradictions inherent in the system—especially the trade-offs between productivity and quality, and energy consumption versus sustainability—will be critical for the widespread adoption of vertical farming as a viable solution for future food production.
To scale vertical farming effectively and meet the growing global demand for food, governments, private investors, and innovators must collaborate to reduce costs, improve energy efficiency, and ensure that the system remains economically and environmentally sustainable. Public-private partnerships, advances in technology, and supportive policy frameworks will be key to overcoming the current barriers and realizing the full potential of vertical farming. By addressing the inherent contradictions and investing in the necessary infrastructure, vertical farming can become a cornerstone of sustainable urban agriculture, ensuring food security and resilience in the face of future global challenges.